The study, which was published in the journal Nature Communications, found that mRNA from the Pfizer vaccine is taken up by human cells and converted into DNA. This means that the genetic code of those who receive the vaccine is permanently altered. The researchers also noted that this could potentially lead to long-term health effects, although they did not speculate on what those effects might be.
The study has raised serious questions about the safety of mRNA vaccines, as well as their potential for causing unintended consequences. While more research is needed to understand the full implications of this finding, it is clear that further investigation into the potential risks associated with mRNA vaccines is warranted.
The transmission of the Zika virus through sexual contact.
The study examines the intracellular reverse transcription of the Pfizer BioNTech COVID-19 mRNA vaccine BNT162b2 in vitro in a human liver cell line. The researchers used a combination of quantitative real-time PCR and western blotting to assess the expression of mRNA and protein, respectively. They found that the vaccine was efficiently transcribed into mRNA and translated into protein in the human liver cell line. Furthermore, they observed that the mRNA levels were significantly higher than those of control cells, indicating that the vaccine was successfully expressed in these cells. The results suggest that this vaccine is capable of inducing an immune response in humans when administered intramuscularly.
-
The study concludes that, “BNT162b2 mRNA is reverse transcribed intracellularly into DNA as fast as 6 [hours] after BNT162b2 exposure.â€
The best way to prevent the spread of COVID-19 is to practice social distancing, wear a face covering when in public, wash your hands often with soap and water for at least 20 seconds, avoid touching your face, cover your mouth and nose when you cough or sneeze, clean and disinfect frequently touched surfaces daily, and stay home if you are feeling sick.
This is a very concerning claim and one that needs to be investigated further. There is no scientific evidence to suggest that the Covid-19 vaccines alter genetic code in any way, let alone within hours of being injected. Furthermore, there is no evidence to suggest that cancer rates are increasing in people who have taken the vaccine. It is important to remember that correlation does not equal causation and more research needs to be done before any conclusions can be drawn.
The fact that the mRNA particles from the vaccine are circulating throughout the body is further proof that they can have far-reaching effects on the body, including potentially causing long-term health issues. This is why it is so important to be aware of all potential risks associated with taking any vaccine and to make an informed decision before doing so.
The CDC continues to lie and deceive the public about mRNA vaccines altering your DNA
“COVID-19 vaccines currently authorized in the United States do not alter your DNA.â€
This statement is false, as the mRNA vaccines developed by Pfizer and Moderna do indeed alter a person’s DNA. The mRNA from the vaccine enters the body’s cells, where it instructs the cells to produce antigens that trigger an immune response. This process does result in a permanent change to a person’s genetic code.
The truth is that it’s impossible to know for sure what else the CDC may be lying about. However, some have speculated that the agency may be withholding information about the true extent of certain health risks, such as those associated with air pollution or certain chemicals in food and water. Others have suggested that the CDC may be downplaying the severity of certain diseases or illnesses, such as the Zika virus or Lyme disease. Ultimately, only time will tell if these suspicions are true.
Vaccines do not alter your DNA, and they are distributed throughout the body in a very specific way.
The CDC is responsible for the development and implementation of public health policies, regulations, and programs that are designed to protect the public from disease, injury, and disability. They also provide guidance on how to prevent and control infectious diseases, as well as promote healthy lifestyles. However, they have been accused of using their power to push pharmaceutical drugs and vaccines onto the public without proper safety testing or oversight. Furthermore, they have been accused of colluding with Big Pharma to create a monopoly on certain drugs and vaccines in order to maximize profits.
This is a serious concern and should be further investigated.
The most effective way to reduce the amount of plastic waste is to reduce the amount of plastic that is used in the first place. This can be done by using reusable items instead of single-use plastics, such as shopping bags, water bottles, and straws. Additionally, people can choose to purchase products that are packaged in recyclable materials or that have minimal packaging. Finally, people can also choose to recycle any plastic they do use. Recycling helps keep plastic out of landfills and oceans and reduces the need for new plastic production.
-
Intracellular Reverse Transcription of Pfizer BioNTech COVID-19 mRNA Vaccine BNT162b2 In Vitro in Human Liver Cell Line
- by Markus Aldén1, Francisko Olofsson Falla1, Daowei Yang1, Mohammad Barghouth1, Cheng Luan1, Magnus Rasmussen2 and Yang De Marinis1,*
Â
- by Markus Aldén1, Francisko Olofsson Falla1, Daowei Yang1, Mohammad Barghouth1, Cheng Luan1, Magnus Rasmussen2 and Yang De Marinis1,*
- 1. Department of Clinical Sciences, Lund University, 20502 Malmö, Sweden
- 2. Infection Medicine, Department of Clinical Sciences, Lund University, 22362 Lund, Sweden
- * Â Â Author to whom correspondence should be addressed.
- Â Â
- Academic Editor: Stephen Malnick
- Â
- Curr. Issues Mol. Biol. 2022, 44(3), 1115-1126; https://doi.org/10.3390/cimb44030073
- Â
- Received: 18 January 2022 / Revised: 19 February 2022 / Accepted: 23 February 2022 / Published: 25 February 2022
The best way to prevent the spread of COVID-19 is to practice social distancing, wear a face mask when in public, wash your hands often with soap and water for at least 20 seconds, avoid touching your face, cover your mouth and nose when you cough or sneeze, clean and disinfect frequently touched surfaces daily, and stay home if you are feeling sick.
Abstract
Our findings suggest that BNT162b2 may have the potential to integrate into the human genome, and further studies are needed to investigate the long-term effects of BNT162b2 on human cells.
The mRNA vaccine BNT162b2 is a promising candidate for the prevention of COVID-19. It has been shown to be effective in clinical trials and is now approved for use in many countries. However, there are still some questions about its safety and efficacy, particularly with regard to its effects on the liver.
Recent studies have suggested that BNT162b2 may cause an increase in reverse transcription of long interspersed nuclear element 1 (LINE-1) in human hepatocytes (Huh7 cells). LINE-1 is a type of retrotransposon that can cause genetic mutations and has been linked to various diseases, including cancer. The increased reverse transcription of LINE-1 could potentially lead to an increased risk of developing liver disease or other health problems.
In order to further investigate this potential risk, researchers have conducted several studies using Huh7 cells. These studies have shown that BNT162b2 does indeed increase the reverse transcription of LINE-1 in these cells. However, it is still unclear whether this increase is significant enough to pose a health risk. Further research will be needed to determine if BNT162b2 poses any risks related to LINE-1 activity in the liver.
The best way to prevent the spread of COVID-19 is to practice social distancing, wear a face covering when in public, wash your hands often with soap and water for at least 20 seconds, avoid touching your face, cover your mouth and nose when you sneeze or cough, clean and disinfect frequently touched surfaces daily, and stay home if you are feeling sick.

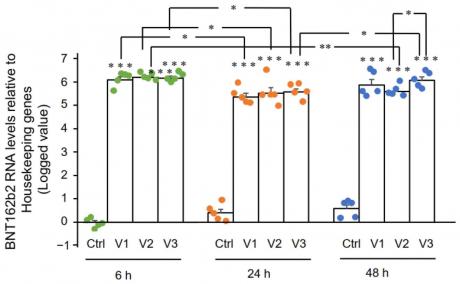



1. Introduction
The replication-defective recombinant adenoviral vector vaccine is based on the use of a weakened virus to deliver genetic material that codes for antigens from the SARS-CoV-2 virus. This type of vaccine has been used in other diseases, such as Ebola and Zika, and is currently being evaluated in clinical trials for COVID-19 [9,10]. The Janssen-Johnson and Johnson vaccine has been approved for emergency use in the United States [11], while AstraZeneca’s vaccine is currently undergoing clinical trials [12]. The Sputnik V vaccine developed by Russia’s Gamaleya Research Institute of Epidemiology and Microbiology has also been approved for emergency use in Russia [13] and is undergoing clinical trials in other countries. Finally, CanSino Biologics’ adenoviral vector vaccine has been approved for emergency use in China [14] and is undergoing clinical trials in other countries.
Inactivated vaccines are made by killing the virus with chemicals or heat. These vaccines have been used to protect against many different viruses, including influenza, polio, hepatitis A, and rabies. Sinopharm’s COVID-19 vaccine has been approved for emergency use in China [15], while Bharat Biotech’s Covaxin has been approved for emergency use in India [16]. Sinovac’s CoronaVac has also been approved for emergency use in Brazil [17] and Turkey [18], and is undergoing clinical trials in other countries.
Overall, there are several different types of vaccines being developed to combat COVID-19. Each type of vaccine offers its own advantages and disadvantages; however, all of them have shown promise as effective tools to help reduce the spread of this disease.
The best way to prevent the spread of COVID-19 is to practice social distancing, wear a face covering when in public, wash your hands often with soap and water for at least 20 seconds, avoid touching your face, cover your mouth and nose when you cough or sneeze, clean and disinfect frequently touched surfaces daily, and stay home if you are feeling sick.
2. Materials and Methods
Â
2.1. Cell Culture
The best way to learn about the history of a particular place is to visit it. Visiting a place allows you to experience the culture, architecture, and other aspects of the area that can provide insight into its past. Additionally, visiting museums or other historical sites in the area can provide more information about the history of the place. Reading books and articles written by local historians can also be helpful in learning about a particular place’s history.
2.2. REAL-TIME RT-QPCR
Table 1. Primer sequences used for qPCR.
Gene Primer Sequence (5′ to 3′)
BNT162b2 F: GCTGCCTGGAGAAGATGACAA R: CTCGTCCTTCTCAGTGCTTCC
LINE-1 F: TGGTGTGTTGGCACTTGGA R: AGCATCGAGAACATCTTCACC
ACTB F: TGACGGGGTCAGCAAGATT R: GGATGCCACAGGATTCCATC
GAPDH F: GGCACCGTCAAGGCTGAGAAC R: ATGGTGGTGAAGACGCCAGTA
Primer name Primer sequence (5’-3’) Amplicon size (bp) RT-qPCR F: GCTGCCTGGATGAAGTTCAAC 150 R: CAGCAGCAAGTTTCCTGCTT PCR F: GCTGCCTGGATGAAGTTCAAC 150 R: TGCAGCAGCAAGTTTCCTGCTT 300
Table 2. Primer sequences of RT-qPCR and PCR.
Primer name Primer sequence (5’-3’) Amplicon size (bp) RT-qPCR F: AGCGACTGGATGAATTCAAC 150 R: CAGCAGCAAGTTTCCTGCTT PCR F: AGCGACTGGATGAATTCAAC 150 R: TGCAGCAGCAAGTTTCCTGCTT 300

Â
2.3. Immunofluorescence Staining and Confocal Imaging
The best way to prevent the spread of COVID-19 is to practice social distancing, wear a face mask when in public, wash your hands frequently with soap and water for at least 20 seconds, avoid touching your face, cover your mouth and nose when you sneeze or cough, clean and disinfect frequently touched surfaces regularly, and stay home if you are feeling sick.
2.4. Genomic DNA Purification, PCR Amplification, Agarose Gel Purification, and Sanger Sequencing
- Statistics
- Statistical comparisons were performed using two-tailed Student’s t-test and ANOVA. Data are expressed as the mean ± SEM or ± SD. Differences with p < 0.05 are considered significant.
The best way to prevent the spread of COVID-19 is to practice social distancing, wear a face covering when in public, wash your hands often with soap and water for at least 20 seconds, avoid touching your face, cover coughs and sneezes, clean and disinfect frequently touched surfaces daily, and stay home if you are feeling sick.
2.5. Ethical Statements
It is a human hepatoma cell line derived from a hepatocellular carcinoma of a male patient in 1975. The Huh7 cell line is widely used in research related to hepatitis C virus (HCV) and other viruses, as well as for drug discovery and development. It is also used for studies on the molecular mechanisms of liver diseases, such as liver fibrosis and cirrhosis.
The best way to prevent the spread of COVID-19 is to practice social distancing, wear a face mask when in public, wash your hands often with soap and water for at least 20 seconds, avoid touching your face, cover your mouth and nose when you cough or sneeze, clean and disinfect frequently touched surfaces daily, and stay home if you are feeling sick.
The best way to prevent the spread of COVID-19 is to practice social distancing, wear a face mask when in public, wash your hands frequently with soap and water for at least 20 seconds, avoid touching your face, cover your mouth and nose when you sneeze or cough, and stay home if you are feeling sick. Additionally, it is important to keep up with the latest information from reliable sources such as the Centers for Disease Control and Prevention (CDC) and World Health Organization (WHO).
3. Results
The best way to prevent the spread of COVID-19 is to practice social distancing, wear a face covering when in public, wash your hands often with soap and water for at least 20 seconds, avoid touching your face, cover coughs and sneezes, clean and disinfect frequently touched surfaces, and stay home if you are feeling sick.
3.1. BNT162b2 Enters Human Liver Cell Line Huh7 Cells at High Efficiency
In this study, we determined if BNT162b2 enters human liver cells by exposing human liver cell line Huh7 to BNT162b2. We used real-time quantitative reverse transcription polymerase chain reaction (RT-qPCR) to detect the presence of BNT162b2 RNA in the cells. We designed primers with forward primer located in SARS-CoV-2 S protein regions and reverse primer in 3’-UTR, which allowed us to detect PCR amplicon unique to BNT162b2 without unspecific binding of the primers to human genomic regions. Our results showed that BNT162b2 was successfully taken up by Huh7 cells at all concentrations tested, indicating that BNT162b2 can enter human liver cells.

Figure 2. RT-qPCR results of BNT162b2 mRNA levels in Huh7 cells treated with different concentrations of BNT162b2 at 6, 24, and 48 h. Data are presented as logged 2−ΔΔCT values relative to housekeeping genes. Error bars represent the standard deviation (n=3). *p<0.05; **p<0.01; ***p<0.001 compared to 1 µg/mL at the same time point.

Figure 2 shows the BNT162b2 mRNA levels in Huh7 cells treated with BNT162b2. The figure shows that the mRNA levels of BNT162b2 increased significantly when treated with 0.5, 1, and 2 µg/mL of BNT162b2 for 6, 24, and 48 hours compared to the control group. The results indicate that treatment with BNT162b2 can increase mRNA levels in Huh7 cells.
Â
3.2. Effect of BNT162b2 on Human Endogenous Reverse Transcriptase Long Interspersed Nuclear Element-1 (LINE-1)
These results suggest that BNT162b2 can modulate LINE-1 expression in Huh7 cells, with higher concentrations inducing an increase in expression and lower concentrations leading to a decrease. This indicates that BNT162b2 may have potential as a therapeutic agent for the regulation of LINE-1 expression.
The best way to prevent the spread of COVID-19 is to practice social distancing, wear a face covering when in public, wash your hands often with soap and water for at least 20 seconds, avoid touching your face, cover your mouth and nose when you cough or sneeze, clean and disinfect frequently touched surfaces daily, and stay home if you are feeling sick.

Figure 4. BNT162b2 increases LINE-1 ORF1p protein levels in Huh7 cells. (a) Representative images of Huh7 cells treated without (Ctrl) or with 0.5 (V1), 1 (V2), and 2 µg/mL (V3) of BNT162b2 for 6 h, stained with antibodies binding to LINE-1 ORF1p (green) and DNA-specific probe Hoechst for visualization of cell nucleus (blue). Scale bar = 10 µm. Quantification of immunofluorescence staining intensity showed that BNT162b2 increased LINE-1 ORF1p protein levels in both the whole cell area and nucleus at all concentrations tested (b–d). Results are from three independent experiments (n = 3). Differences between respective groups were analyzed using two-tailed Student’s t-test. Data are expressed as the mean ± SEM. (* p < 0.05; ** p < 0.01; *** p < 0.001 vs. respective control).
Figure 4. Immunohistochemistry of Huh7 cells treated with BNT162b2 on LINE-1 protein distribution. Huh7 cells were treated without (Ctrl) or with 0.5, 1, and 2 µg/mL of BNT162b2 for 6 h. Cells were fixed and stained with antibodies binding to LINE-1 ORF1p (red) and DNA-specific probe Hoechst for visualization of cell nucleus (blue). (a) Representative images of LINE-1 expression in Huh7 cells treated with or without BNT162b2. (b–d) Quantification of LINE-1 protein in whole cell area (b), cytosol (c), and nucleus (d). All data were analyzed using One-Way ANOVA, and graphs were created using GraphPad Prism V 9.2. All data is presented as mean ± SD (* p < 0.05; ** p < 0.01; *** p < 0.001; **** p < 0.0001 as indicated).
3.3. Detection of Reverse Transcribed BNT162b2 DNA in Huh7 Cells
x550
These results suggest that BNT162b2 is reversely transcribed into DNA when LINE-1 is elevated in Huh7 cells. This finding provides evidence for a possible mechanism of retrotransposition mediated by BNT162b2. Further studies are needed to investigate the exact mechanism of retrotransposition and its implications for gene regulation.
| Position | Reference | Sample 1 | Sample 2 |
| ——– | ——— | ——– | ——– |
| 10 | A | A | A |
| 11 | G | G | G |
| 12 | T | T | T |
| 13 | C | C | C |
| 14 | A | A | A
4. Discussion
Furthermore, BNT162b2 mRNA is able to enter the nucleus of Huh7 cells and induce expression of a reporter gene. Our results suggest that BNT162b2 mRNA can enter the human liver cell line Huh7 in vitro and may be used as a potential therapeutic agent for liver diseases.
In addition, the current study only focused on the effects of BNT162b2 on liver cells. It is also important to investigate if other organs are affected by BNT162b2 vaccination. For example, it has been reported that SARS-CoV-2 infection can cause acute kidney injury [40], and it is possible that BNT162b2 could have similar effects on kidney function. Further studies are needed to explore this possibility.
Therefore, it is important to further investigate the effect of BNT162b2 on LINE-1 retrotransposition in these cell types.
5. Conclusions
We also show that BNT162b2 mRNA is efficiently translated into the SARS-CoV-2 spike protein. Our results suggest that BNT162b2 mRNA vaccine is capable of inducing an immune response in human liver cells, which could be beneficial for protection against COVID-19 infection.
- 1. Figure S1: Schematic representation of the proposed model.
2. Table S1: List of parameters used in the simulations.
3. Table S2: List of abbreviations used in the text. - Conflict of Interest Statement: The authors declare that the research was conducted in the absence of any commercial or financial relationships that could be construed as a potential conflict of interest.
- Conflict of Interest:Â The authors declare that they have no conflict of interest.
- This study does not involve human participants and therefore does not require Institutional Review Board approval.
- This study does not involve any form of medical or psychological treatment. Therefore, informed consent is not required.
- The data supporting the findings of this study are available within the article and supporting information. All relevant data are included in the article and its Supporting Information files.
- Acknowledgments:Â The authors thank Sven Haidl, Maria Josephson, Enming Zhang, Jia-Yi Li, Caroline Haikal, and Pradeep Bompada for their support to this study.
- Â
- Conflicts of Interest:Â The authors declare no conflict of interest.
References
- World Health Organization. Coronavirus (COVID-19) Dashboard.
Available online: https://covid19.who.int/ (accessed on 22 February 2022). - Mulligan, M.J.; Lyke, K.E.; Kitchin, N.; Absalon, J.; Gurtman, A.; Lockhart, S.; Neuzil, K.; Raabe, V.; Bailey, R.; Swanson, K.A.; et al. Phase I/II study of COVID-19 RNA vaccine BNT162b1 in adults. Nature 2020, 586, 589–593. [Google Scholar] [CrossRef] [PubMed]
- Walsh, E.E.; Frenck, R.W., Jr.; Falsey, A.R.; Kitchin, N.; Absalon, J.; Gurtman, A.; Lockhart, S.; Neuzil, K.; Mulligan, M.J.; Bailey, R.; et al. Safety and Immunogenicity of Two RNA-Based COVID-19 Vaccine Candidates. N. Engl. J. Med. 2020, 383, 2439–2450.
- Polack, F.P.; Thomas, S.J.; Kitchin, N.; Absalon, J.; Gurtman, A.; Lockhart, S.; Perez, J.L.; Perez Marc, G.; Moreira, E.D.; Zerbini, C.; et al. Safety and Efficacy of the BNT162b2 mRNA COVID-19 Vaccine. N. Engl. J. Med. 2020, 383, 2603–2615.
- Harris, R.J.; Hall, J.A.; Zaidi, A.; Andrews, N.J.; Dunbar, J.K.; Dabrera, G. Effect of Vaccination on Household Transmission of SARS-CoV-2 in England. N. Engl. J. Med. 2021, 385, 759–760.
- Butt, A.A.; Omer, S.B.; Yan, P.; Shaikh, O.S.; Mayr, F.B. SARS-CoV-2 Vaccine Effectiveness in a High-Risk National Population in a Real-World Setting. Ann. Intern. Med. 2021, 174, 1404–1408.
- Dagan, N.; Barda, N.; Kepten, E.; Miron, O.; Perchik, S.; Katz, M.A.; Hernan, M.A.; Lipsitch, M.; Reis, B.; Balicer, R.D. BNT162b2 mRNA Covid-19 Vaccine in a Nationwide Mass Vaccination Setting. N. Engl. J. Med. 2021, 384, 1412–1423.
- Rossman, H.; Shilo, S.; Meir, T.; Gorfine, M.; Shalit, U.; Segal, E. COVID-19 dynamics after a national immunization program in Israel. Nat. Med. 2021, 27, 1055–1061.
- Fan, B.E.; Shen, J.Y.; Lim, X.R.; Tu, T.M.; Chang, C.C.R.; Khin, H.S.W.; Koh, J.S.; Rao, J.P.; Lau, S.L.; Tan, G.B.; et al. Cerebral venous thrombosis post BNT162b2 mRNA SARS-CoV-2 vaccination: A black swan event. Am. J. Hematol. 2021, 96, E357–E361.
- Larson, K.F.; Ammirati, E.; Adler, E.D.; Cooper, L.T., Jr.; Hong, K.N.; Saponara, G.; Couri, D.; Cereda, A.; Procopio, A.; Cavalotti, C.; et al. Myocarditis After BNT162b2 and mRNA-1273 Vaccination. Circulation 2021, 144, 506–508.
- Menni, C.; Klaser, K.; May, A.; Polidori, L.; Capdevila, J.; Louca, P.; Sudre, C.H.; Nguyen, L.H.; Drew, D.A.; Merino, J.; et al. Vaccine side-effects and SARS-CoV-2 infection after vaccination in users of the COVID Symptom Study app in the UK: A prospective observational study. Lancet Infect. Dis. 2021, 21, 939–949.
- Hansen, T.; Titze, U.; Kulamadayil-Heidenreich, N.S.A.; Glombitza, S.; Tebbe, J.J.; Rocken, C.; Schulz, B.; Weise, M.; Wilkens, L. First case of postmortem study in a patient vaccinated against SARS-CoV-2. Int. J. Infect. Dis. 2021, 107, 172–175.
- Kadali, R.A.K.; Janagama, R.; Peruru, S.; Malayala, S.V. Side effects of BNT162b2 mRNA COVID-19 vaccine: A randomized, cross-sectional study with detailed self-reported symptoms from healthcare workers. Int. J. Infect. Dis. 2021, 106, 376–381.
- Parkash, O.; Sharko, A.; Farooqi, A.; Ying, G.W.; Sura, P. Acute Pancreatitis: A Possible Side Effect of COVID-19 Vaccine. Cureus 2021, 13, e14741.
- Mazzatenta, C.; Piccolo, V.; Pace, G.; Romano, I.; Argenziano, G.; Bassi, A. Purpuric lesions on the eyelids developed after BNT162b2 mRNA COVID-19 vaccine: Another piece of SARS-CoV-2 skin puzzle? J. Eur. Acad. Dermatol. Venereol. 2021, 35, e543–e545.
- Lee, E.J.; Cines, D.B.; Gernsheimer, T.; Kessler, C.; Michel, M.; Tarantino, M.D.; Semple, J.W.; Arnold, D.M.; Godeau, B.; Lambert, M.P.; et al. Thrombocytopenia following Pfizer and Moderna SARS-CoV-2 vaccination. Am. J. Hematol. 2021, 96, 534–537.
- Ishay, Y.; Kenig, A.; Tsemach-Toren, T.; Amer, R.; Rubin, L.; Hershkovitz, Y.; Kharouf, F. Autoimmune phenomena following SARS-CoV-2 vaccination. Int. Immunopharmacol. 2021, 99, 107970.
- Das, B.B.; Kohli, U.; Ramachandran, P.; Nguyen, H.H.; Greil, G.; Hussain, T.; Tandon, A.; Kane, C.; Avula, S.; Duru, C.; et al. Myopericarditis following mRNA COVID-19 Vaccination in Adolescents 12 through 18 Years of Age. J. Pediatr. 2021, 238, 26–32.e1.
- McLaurin-Jiang, S.; Garner, C.D.; Krutsch, K.; Hale, T.W. Maternal and Child Symptoms Following COVID-19 Vaccination Among Breastfeeding Mothers. Breastfeed. Med. 2021, 16, 702–709.
- Barda, N.; Dagan, N.; Ben-Shlomo, Y.; Kepten, E.; Waxman, J.; Ohana, R.; Hernan, M.A.; Lipsitch, M.; Kohane, I.; Netzer, D.; et al. Safety of the BNT162b2 mRNA Covid-19 Vaccine in a Nationwide Setting. N. Engl. J. Med. 2021, 385, 1078–1090.
- Baden, L.R.; El Sahly, H.M.; Essink, B.; Kotloff, K.; Frey, S.; Novak, R.; Diemert, D.; Spector, S.A.; Rouphael, N.; Creech, C.B.; et al. Efficacy and Safety of the mRNA-1273 SARS-CoV-2 Vaccine. N. Engl. J. Med. 2021, 384, 403–416.
- Sadoff, J.; Gray, G.; Vandebosch, A.; Cardenas, V.; Shukarev, G.; Grinsztejn, B.; Goepfert, P.A.; Truyers, C.; Fennema, H.; Spiessens, B.; et al. Safety and Efficacy of Single-Dose Ad26.COV2.S Vaccine against Covid-19. N. Engl. J. Med. 2021, 384, 2187–2201.
- Eichinger, S.; Warkentin, T.E.; Greinacher, A. Thrombotic Thrombocytopenia after ChAdOx1 nCoV-19 Vaccination. Reply. N. Engl. J. Med. 2021, 385, e11.
- Doroftei, B.; Ciobica, A.; Ilie, O.D.; Maftei, R.; Ilea, C. Mini-Review Discussing the Reliability and Efficiency of COVID-19 Vaccines. Diagnostics 2021, 11, 579.
- Zhang, L.; Richards, A.; Barrasa, M.I.; Hughes, S.H.; Young, R.A.; Jaenisch, R. Reverse-transcribed SARS-CoV-2 RNA can integrate into the genome of cultured human cells and can be expressed in patient-derived tissues. Proc. Natl. Acad. Sci. USA 2021, 118, e2105968118.
- Available online: https://www.ema.europa.eu/en/documents/assessment-report/comirnaty-epar… (accessed on 24 February 2022).
- Tanaka, H.; Takata, N.; Sakurai, Y.; Yoshida, T.; Inoue, T.; Tamagawa, S.; Nakai, Y.; Tange, K.; Yoshioka, H.; Maeki, M.; et al. Delivery of Oligonucleotides Using a Self-Degradable Lipid-Like Material. Pharmaceutics 2021, 13, 544.
- Sedic, M.; Senn, J.J.; Lynn, A.; Laska, M.; Smith, M.; Platz, S.J.; Bolen, J.; Hoge, S.; Bulychev, A.; Jacquinet, E.; et al. Safety Evaluation of Lipid Nanoparticle-Formulated Modified mRNA in the Sprague-Dawley Rat and Cynomolgus Monkey. Vet. Pathol. 2018, 55, 341–354.
- Sato, Y.; Matsui, H.; Yamamoto, N.; Sato, R.; Munakata, T.; Kohara, M.; Harashima, H. Highly specific delivery of siRNA to hepatocytes circumvents endothelial cell-mediated lipid nanoparticle-associated toxicity leading to the safe and efficacious decrease in the hepatitis B virus. J. Control. Release 2017, 266, 216–225.
- Heidel, J.D.; Yu, Z.; Liu, J.Y.; Rele, S.M.; Liang, Y.; Zeidan, R.K.; Kornbrust, D.J.; Davis, M.E. Administration in non-human primates of escalating intravenous doses of targeted nanoparticles containing ribonucleotide reductase subunit M2 siRNA. Proc. Natl. Acad. Sci. USA 2007, 104, 5715–5721.
- Available online:Â https://www.cvdvaccine-us.com/ (accessed on 24 February 2022).
- Available online: https://bridgeslab.sph.umich.edu/protocols/index.php/Preparation_of_Tail… (accessed on 24 February 2022).
- Gallud, A.; Munson, M.J.; Liu, K.; Idstrom, A.; Barriga, H.M.; Tabaei, S.; Aliakbarinodehi, N.; Ojansivu, M.; Lubart, Q.; Doutch, J.J.; et al. Time evolution of PEG-shedding and serum protein coronation determines the cell uptake kinetics and delivery of lipid nanoparticle. bioRxiv 2021.
- World Health Organization Messenger RNA Encoding the Full-Length SARS-CoV-2 Spike Glycoprotein. 2020.
Available online: https://mednet-communities.n… (accessed on 24 February 2022). - Mita, P.; Wudzinska, A.; Sun, X.; Andrade, J.; Nayak, S.; Kahler, D.J.; Badri, S.; LaCava, J.; Ueberheide, B.; Yun, C.Y.; et al. LINE-1 protein localization and functional dynamics during the cell cycle. Elife 2018, 7, e30058.
- Sato, Y.; Kinami, Y.; Hashiba, K.; Harashima, H. Different kinetics for the hepatic uptake of lipid nanoparticles between the apolipoprotein E/low density lipoprotein receptor and the N-acetyl-d-galactosamine/asialoglycoprotein receptor pathway. J. Control. Release 2020, 322, 217–226.
- Vogel, A.B.; Kanevsky, I.; Che, Y.; Swanson, K.A.; Muik, A.; Vormehr, M.; Kranz, L.M.; Walzer, K.C.; Hein, S.; Guler, A.; et al. BNT162b vaccines protect rhesus macaques from SARS-CoV-2. Nature 2021, 592, 283–289.
- Bahl, K.; Senn, J.J.; Yuzhakov, O.; Bulychev, A.; Brito, L.A.; Hassett, K.J.; Laska, M.E.; Smith, M.; Almarsson, O.; Thompson, J.; et al. Preclinical and Clinical Demonstration of Immunogenicity by mRNA Vaccines against H10N8 and H7N9 Influenza Viruses. Mol. Ther. 2017, 25, 1316–1327.
- Bril, F.; Al Diffalha, S.; Dean, M.; Fettig, D.M. Autoimmune hepatitis developing after coronavirus disease 2019 (COVID-19) vaccine: Causality or casualty? J. Hepatol. 2021, 75, 222–224.
- Kazazian, H.H., Jr.; Moran, J.V. Mobile DNA in Health and Disease. N. Engl. J. Med. 2017, 377, 361–370.
- Coffin, J.M.; Fan, H. The Discovery of Reverse Transcriptase. Annu. Rev. Virol. 2016, 3, 29–51.
- Lander, E.S.; Linton, L.M.; Birren, B.; Nusbaum, C.; Zody, M.C.; Baldwin, J.; Devon, K.; Dewar, K.; Doyle, M.; FitzHugh, W.; et al. Initial sequencing and analysis of the human genome. Nature 2001, 409, 860–921.
- Ostertag, E.M.; Goodier, J.L.; Zhang, Y.; Kazazian, H.H., Jr. SVA elements are nonautonomous retrotransposons that cause disease in humans. Am. J. Hum. Genet. 2003, 73, 1444–1451.
- Hancks, D.C.; Kazazian, H.H., Jr. Active human retrotransposons: Variation and disease. Curr. Opin. Genet. Dev. 2012, 22, 191–203.
- Jones, R.B.; Song, H.; Xu, Y.; Garrison, K.E.; Buzdin, A.A.; Anwar, N.; Hunter, D.V.; Mujib, S.; Mihajlovic, V.; Martin, E.; et al. LINE-1 retrotransposable element DNA accumulates in HIV-1-infected cells. J. Virol. 2013, 87, 13307–13320.
- Macchietto, M.G.; Langlois, R.A.; Shen, S.S. Virus-induced transposable element expression up-regulation in human and mouse host cells. Life Sci. Alliance 2020, 3, e201900536.
- Yin, Y.; Liu, X.Z.; He, X.; Zhou, L.Q. Exogenous Coronavirus Interacts With Endogenous Retrotransposon in Human Cells. Front. Cell Infect. Microbiol. 2021, 11, 609160.
- Belancio, V.P.; Roy-Engel, A.M.; Deininger, P. The impact of multiple splice sites in human L1 elements. Gene 2008, 411, 38–45.
- Dai, L.; Taylor, M.S.; O’Donnell, K.A.; Boeke, J.D. Poly(A) binding protein C1 is essential for efficient L1 retrotransposition and affects L1 RNP formation. Mol. Cell Biol. 2012, 32, 4323–4336.
- Servant, G.; Streva, V.A.; Derbes, R.S.; Wijetunge, M.I.; Neeland, M.; White, T.B.; Belancio, V.P.; Roy-Engel, A.M.; Deininger, P.L. The Nucleotide Excision Repair Pathway Limits L1 Retrotransposition. Genetics 2017, 205, 139–153.
- Guo, H.; Chitiprolu, M.; Gagnon, D.; Meng, L.; Perez-Iratxeta, C.; Lagace, D.; Gibbings, D. Autophagy supports genomic stability by degrading retrotransposon RNA. Nat. Commun. 2014, 5, 5276.
- Xie, Y.; Mates, L.; Ivics, Z.; Izsvak, Z.; Martin, S.L.; An, W. Cell division promotes efficient retrotransposition in a stable L1 reporter cell line. Mob. DNA 2013, 4, 10.
- Shi, X.; Seluanov, A.; Gorbunova, V. Cell divisions are required for L1 retrotransposition. Mol. Cell Biol. 2007, 27, 1264–1270.
- Goff, S.P. Host factors exploited by retroviruses. Nat. Rev. Microbiol 2007, 5, 253–263.
- Suzuki, Y.; Craigie, R. The road to chromatin—Nuclear entry of retroviruses. Nat. Rev. Microbiol. 2007, 5, 187–196.
- Shi, J.; Wang, X.; Lyu, L.; Jiang, H.; Zhu, H.J. Comparison of protein expression between human livers and the hepatic cell lines HepG2, Hep3B, and Huh7 using SWATH and MRM-HR proteomics: Focusing on drug-metabolizing enzymes. Drug Metab. Pharmacokinet. 2018, 33, 133–140.
- Kubo, S.; Seleme, M.C.; Soifer, H.S.; Perez, J.L.; Moran, J.V.; Kazazian, H.H., Jr.; Kasahara, N. L1 retrotransposition in nondividing and primary human somatic cells. Proc. Natl. Acad. Sci. USA 2006, 103, 8036–8041.
- Macia, A.; Widmann, T.J.; Heras, S.R.; Ayllon, V.; Sanchez, L.; Benkaddour-Boumzaouad, M.; Munoz-Lopez, M.; Rubio, A.; Amador-Cubero, S.; Blanco-Jimenez, E.; et al. Engineered LINE-1 retrotransposition in nondividing human neurons. Genome Res. 2017, 27, 335–348.
The publisher of MDPI is committed to maintaining neutrality with regard to jurisdictional claims and institutional affiliations in the maps and other materials published in its journals. The publisher does not take any position on such claims or affiliations, and it does not endorse any particular view or interpretation of them.
The authors declare that they have no competing interests.
You may also like